One of the most enduring and polarizing debates in the online community of paleontology enthusiasts is the one about which species should hold the title of the largest (known) theropod, intricately linked to that of the largest terrestrial carnivore of all time. I have been involved in this debate many times over the years, and have some thoughts and opinions that I want to present here in as concise a form as possible. While legitimate arguments might be had in support of several other taxa of theropods, including Spinosaurus, Mapusaurus, Tyrannotitan, Carcharodontosaurus and even, possibly, Allosaurus sp., in recent years this debate has often boiled down to a comparison between Tyrannosaurus rex and its most traditional challenger, Giganotosaurus carolinii. It is hence the comparison between these two taxa that I want to focus on here, bearing in mind that several other giant Carcharodontosaurids might well have had similar average sizes to Giganotosaurus, but are fraught with even greater uncertainty.
T. rex is certainly the best-known, most popular and well-studied contender for the largest theropod dinosaur. Known from dozens of substantial skeletons (e.g. Mortimer 2025 [online], Paul et al. 2022, Larson [et al.] 2008]) it sports a fossil record of a quality and quantity not matched by any other theropod species that might legitimately deserve the label of "giant". Accordingly, it should not come as a surprise that the specimens that are probably the largest known individuals of any theropod are referred to T. rex. Most famously, this includes the two similar-sized and well-preserved skeletons Sue (FMNH PR 2081, femur circumference 580 mm) and Scotty (RSM P2523.8, femur circumference 590 mm), even though they both appear to be outclassed by a less well-known and less complete specimen, BHI 6248 (nicknamed Cope, femur circumference 630 mm). It is at least likely that the latter constitutes the largest theropod specimen currently described, with its femur circumference suggesting a body mass potentially 28% greater than that of Sue. Sue itself measures 12.3 to 12.4 m in total length (Paes 2016 [online], Hutchinson et al. 2011) and has variously been estimated at 8.4 t to 9.5 t (Snively et al. 2019, Hartman 2013 [online]), Bruñén 2016a, 2017 [online], Hutchinson et al. 2011) in mass, already comparable to several of the largest specimens of other theropods (e.g. Hartman 2013 [online], Folkes 2023 [online]) and significantly outclassing individuals that are reasonably complete (e.g. Snively et al. 2019, Campione et al. 2014). For BHI 6248, this suggests a plausible body mass range of 10.7-12 t. Based on this, T. rex is often simply assumed, ex- or implicitly, to have been the largest theropod, and claims to that title made by other taxa in the 90s and early 2000s have largely been ignored or dismissed on these grounds alone.
However, to focus on the size of the largest known individuals is to commit a fundamental statistical fallacy; Most other giant theropods are known from only a handful of specimens at most, and even these are often fragmentary. This makes it unlikely to recover individuals at the extremes of the size distribution for any of these taxa, and any comparison that is based on individual specimens that do not reflect the average size of the taxon is mere cherry-picking. The relevant metric for comparison between T. rex and other animals is not its maximum size (which is not even known and can only be speculated on, see Mallon and Hone 2024), but rather its average size. A caveat to this comparison lies in the inclusion of immature specimens. However, for most relevant taxa we know, or can reasonably assume, which specimens are adult (sexually mature), and can thus reasonably limit our comparisons to specimens that fulfill this criterium. A possible exception is Mapusaurus, known only from a bonebed representing multiple ontogenetic stages over an indeterminate number of total individuals. Since our present comparison is focused on Giganotosaurus, whose holotype is a mature individual (Coria and Currie 2003), we can safely limit our comparison to adults only. In doing that, we find that the average adult T. rex, based on a sample of 33 individuals with known femur length and circumference, or other material permitting their estimation, has a femur length of 1230 mm and a femur circumference of 521 mm. Contrary to common talking points, these values can be estimated fairly accurately (90% CI of femur length 1203-1249 mm, 90% CI of femur circumference 509-533 mm), demonstrating that the sample size is sufficient to estimate average size with a reasonable degree of accuracy.
Circumference | Comment, Source | |
MUCPv-Ch1 | 521 mm | measurement, Campione et al. 2014 |
MUCPv-95 | 533 mm 548 mm 555 mm 563 mm | 2.2% larger minimum dentary depth, Coria and Currie 2006 5.2% larger centroid size (conservative) 6.6% longer alveolar margin, Folkes 2023 [online] 8% larger in unspecified measurement, Calvo and Coria 1998 |
Since its unveiling to the public in 1995, Giganotosaurus carolinii has been recognized as one of the largest known theropods (Coria and Salgado 1995, Calvo and Coria 1998, Mazzetta et al. 2004, Persons et al. 2019), if not the largest. Giganotosaurus is known from only two individuals, one of which is a partial dentary (MUCPv-95, Calvo and Coria 1998) measuring approximately 61 cm in length, while the smaller holotype specimen (MUCPv-Ch1, Coria and Salgado 1995) is a relatively complete skeleton (albeit not on the same level of completeness as Sue, and mostly undescribed as of now) including substantial parts of the skull, vertebral collumn and hindlimb, including a femur with a circumference of 521 mm (Campione et al. 2014), which puts it squarely within T. rex size range. The precise size difference between the two specimens has been a matter of contention: While MUCPv-95 is universally recognized as the larger specimen, estimates have variously placed it between 2% and 8% larger than the holotype specimen. This issue is caused and complicated by the lack of detail in the original descriptions of both specimens, including Calvo and Coria (1998), despite their emphasis on highlighting MUCPv-95 as 8% larger than MUCPv-Ch1, (without giving any specifics or comparative measurements). The most recent information comes from a private communication to Dan Folkes (2023 [online]), and reports that the length taken along the alveoli is 6.6% larger in MUCPv-95. This closely matches the 6.5% estimate by Hartman (2013 [online]), which can be taken as independent verification, and is also plausibly close to my own conservative estimate based on centroid size. 6.6%, despite being unpublished, thus appears as the most relevant and reliable figure to use.
Due to incompleteness and the limited amount of descriptive data on the holotype, the overall body dimensions and mass of Giganotosaurus have likewise been variously estimated. Previous estimates of total length vary from 12.2 to 12.7 m (not counting an estimate of 13 m by Therrien and Henderson 2007, which was only based on estimated skull length and ignored all available postcranial material).
Total Length | Comment, Source | |
MUCPv-Ch1 | 12.2 m 12.3 m 12.4 m 12.5 m 12.7 m | Coria and Currie 2006 Bruñén 2016b [online] Hartman 2013 [online] SpinoInWonderland 2023 [online], Coria and Salgado 1995 Folkes 2023, Paul 2024 |
MUCPv-95 | 12.5–13.0 m 12.8-13.4 13.0-13.5 m 13.2-13.7 | 2.2% larger minimum dentary depth, Coria and Currie 2006 5.2% larger centroid size (conservative) 6.6% longer alveolar margin, Folkes 2023 [online] 8% larger in unspecified measurement, Calvo and Coria 1998 |
Already we can see that Giganotosaurus more than rivals T. rex, and not just in terms of total length. If we compare femur circumferences, a measurement with a strong functional linkage to body mass, even the smaller of the two known Giganotosaurus individuals already matches the average femur circumference of T. rex across 33 specimens. The second, larger specimen shows that this is unlikely to be an unusually large individual of its species, which already suggests that Giganotosaurus is likely at least as large as T. rex, if not larger, since both its known specimens squarely fall into the upper half of T. rex’ size distribution. Assuming MUCPv-95 is 6.6% larger than MUCPv-Ch1, its femur circumference is 555 mm, and the mean circumference for Giganotosaurus becomes 538 mm, which is above the 90% confidence range for the average femur circumference of T. rex. This suggests that Giganotosaurus carolinii is probably the larger species, at least in terms of average size (with comparisons of maximum size being impossible at this time).
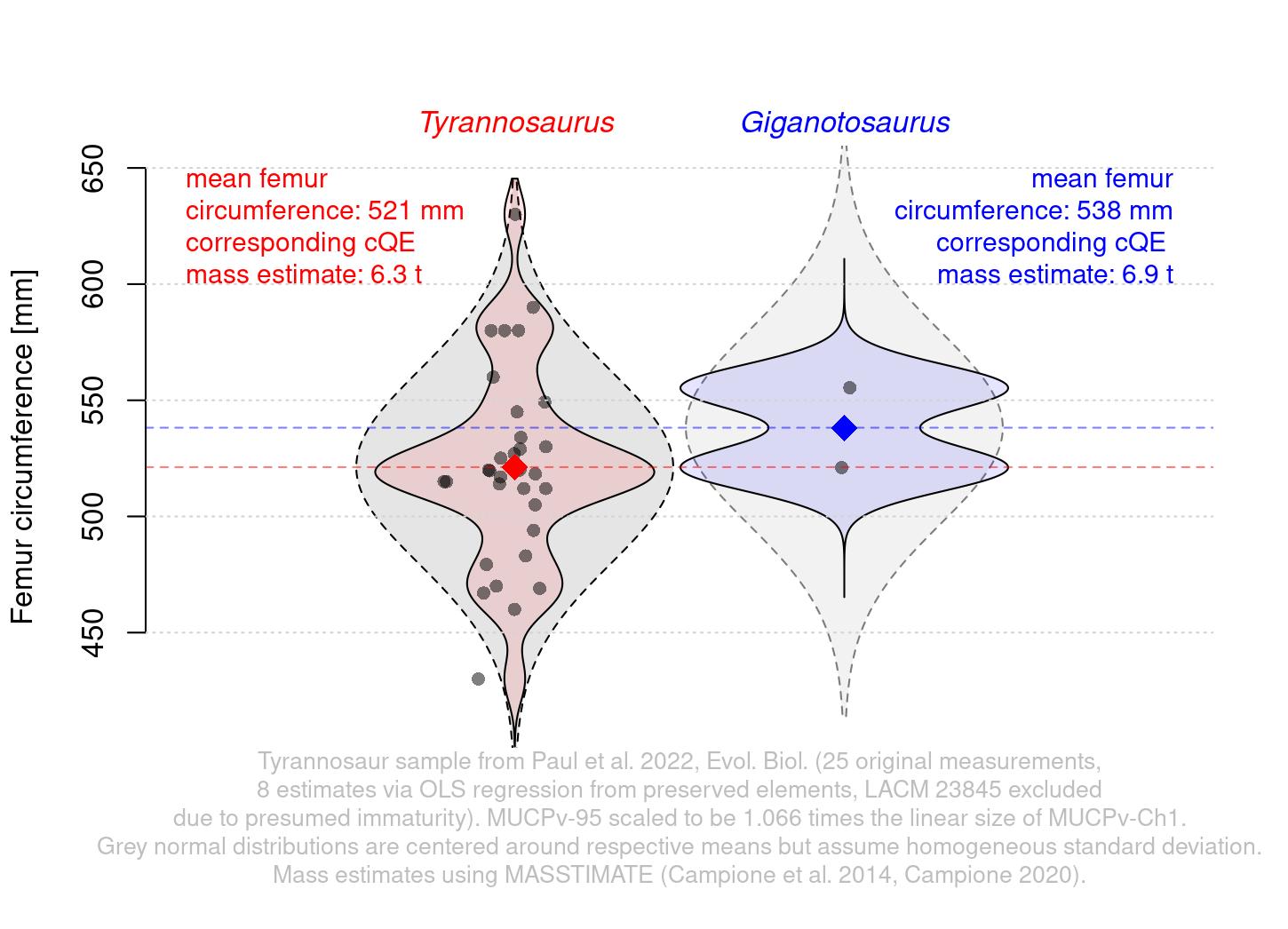
Comparison of the distribution of femur circumferences in T. rex and Giganotosaurus carolinii, with a hypothetical normal distribution of equal variance to that observed in T. rex, but centered on the mean of Giganotosaurus "subimposed" for the Giganotosaurus sample.
We can approach this more quantitatively and estimate the significance level of the apparent size difference, and the power level (using the pwr package in R):
t.test(femur_circumference~taxon, dataset, alternative="greater")
wilcox.test(femur_circumference~taxon, dataset, alternative="greater")
require(pwr)
coh_d<-abs(diff(tapply(dataset$femur_circumference,dataset$taxon,FUN=mean)))/sd(dataset$femur_circumference)
pwr::pwr.t.test(n=2,d=coh_d,power=NULL,sig.level=0.05,alternative="greater")
R code
We observe that the p-value (probability of type I error, i.e. a false positive/false rejection of the null) from the wilcox-test is approximately 0.17 and that from the t-test 0.25, suggesting a non-significant difference in femur circumference between the two taxa. However, assuming accurate estimates of femur circumference, this still means that the probability of the obeserved differences being merely due to chance is <25%, which at least qualifies as a strong tendency by dinosaur standards. Conversely, this means that the observed difference not being due to chance is >75%, i.e. a 75% probability of Giganotosaurus being (at least slightly) larger than T. rex. While there is of course valid reasoning for dismissing a difference that does not meet agreed-upon standards for statistical significance, defaulting to p<0.05, it is worth questioning it p<0.05 is generally a realistic significance level in comparisons between dinosaurs or other poorly sampled extinct taxa. The results of a power analysis illustrate this (statistical power is the probability of type II error, i.e. a false negative/false rejection of the alternative hypothesis): For a cohen’s d of 0.417 (the difference in means divided by pooled standard deviation) as in our case, the statistical power for rejecting the null hypothesis at p<0.05 is just 0.09, i.e. there would have been only a 9% chance of achieving a statistically significant result given that the difference observed in our samples actually reflects the difference in the real populations. In order to achieve a considerably higher power, we would need to either assume a much larger actual difference (e.g. with an effect size of 2 sds, which would be equivalent to a femur circumference difference of over 8 cm, we would achieve a power of 0.38, i.e. a 38% chance of achieving a significant result), higher sample size or higher critical p-value. Since a much larger difference in size between the taxa is not parsimonious to expect (and is not suggested here), and we have to work with the sample sizes that we have, the only option is to critically reconsider our appropriate p-value. In this case, the power analysis indicates that in order to stand a >50% chance of avoiding a false negative, we would have to increase our critical p-value to 0.34, i.e. roughly a third, which I would suggest could be a reasonable rule of thumb for fossil interspecies comparisons where there is no strong mechanistic argument for favoring the null-hypothesis of no difference.
We can thus estimate that the difference in body mass based on femur circumference was likely around 10%, and further illustrate that by plotting the samples of estimated body masses (Using Campione et al.’s mathematically corrected regression equation, which tends to give plausible body mass estimates for T. rex, albeit on the low end of volumetric figures for the same specimens):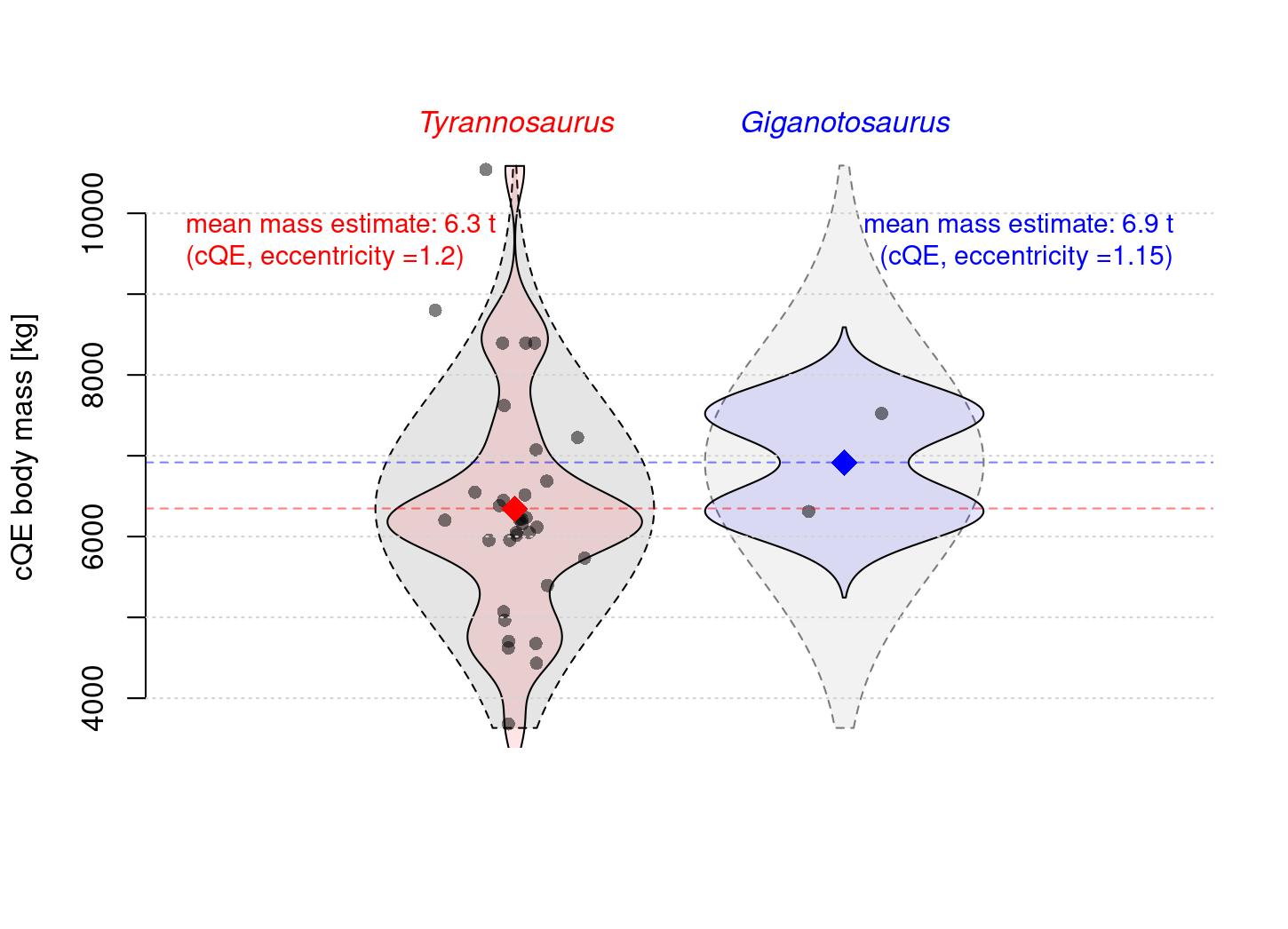
Comparison of the distribution of body mass in T. rex and Giganotosaurus carolinii as based on the results of the phylogenetically corrected cQE (Campione 2020, Campione et al. 2014), with a hypothetical normal distribution of equal variance to that observed in T. rex, but centered on the mean of Giganotosaurus "subimposed" for the Giganotosaurus sample. For the sake of simplicity, all T. rex specimens are assumed to have a femoral eccentricity of 1.2, whereas for Giganotosaurus the exact eccentricity (approx. 1.15) of MUCPv-Ch1 is used.
What does all this imply? It means we cannot be highly confident that Giganotosaurus was larger than Tyrannosaurus, but we can say that it is likely that it was – or at least decidedly more likely than for the reverse to be true. I would argue that in vertebrate paleontology, such a tendency is generally sufficient to establish a difference, because otherwise many common-sense interspecific comparisons are suddenly rendered impossible due to small sample sizes – even ones as obvious and clear-cut as that between Argentinosaurus and Epidexipteryx (which are both only known from a single specimen, thus precluding an estimation of the statistical significance of their sizen difference). In any case, it is certainly a higher significance level than any (implicit or explicit) usage of individually picked specimens can provide (it is often ignored that if even a comparison of species averages fails to achieve sufficient significance, then any comparison between single specimens is by necessity even less meaningful). There are also reasons to think that the present comparison based on femur circumference is more likely than not to underestimate the degree to which Giganotosaurus is larger. Notably, known Tyrannosaurus specimens tend to have robust femora for their (volumetrically estimated) body mass. Famously, the T. rex specimen USNM 555 has a femur circumference of 520 mm, while the Acrocanthosaurus specimen NCSM 14345 has a femur circumference of 427 mm (Campione et al. 2014). Based on this, the former specimen would be predicted to be almost twice the mass of the latter, but volumetric studies of the complete specimens suggest that the two are actually very similar in mass (Bates et al. 2009). Volumetric estimates for Giganotosaurus show a similar, albeit less extreme, trend, suggesting the larger Giganotosaurus specimen to be, volumetrically, at least on par with T. rex specimens that have femur circumferences several cm greater than its own:
Body Mass | Specific Gravity, Source | |
MUCPv-Ch1 | 6.8 t 6.9 t 7.4 t 8 t 8.2 t 8.8 t | SG 0.91, Hartman 2013 [online] unspecified SG, Snively et al. 2019 SG 0.97, Bruñén 2016b [online] SG ?0.97, Paul 2024 SG 0.95, SpinoInWonderland 2022 [online] SG 0.97, Folkes 2023 |
MUCPv-95 | 7.3–9.4 t 7.9–10.2 t 8.2–10.7 t 8.6–11.1 t | 2.2% larger minimum dentary depth, Coria and Currie 2006 5.2% larger centroid size (conservative) 6.6% longer alveolar margin, Folkes 2023 [online] 8% larger in unspecified measurement, Calvo and Coria 1998 |
Volumetric mass estimates consistently show that an upscaled MUCPv-95 (at ≥6.5% larger linearly than MUCPv-Ch1) would at least rival (e.g. Hartman 2013 [online], Bruñén 2016ab [online], Snively et al. 2019), if not exceed (SpinoInWonderland 2022 [online], Folkes 2023 [online], Paul 2024) the mass of T. rex specimens like Sue or Scotty (note the non-independent effects of the assumed density, which ranges from 0.91 to 0.97 for different estimates, the latter based on Larramendi et al. 2021).
One concern that is sometimes raised is the inclusivity of the T. rex sample used herein, specifically with the assertion that the known Giganotosaurus specimens should only be compared to those T. rex individuals that have an external fundamental system (EFS) or other clear indications of being "asymptotic" (i.e in the asymptotic growth stage sensu Erickson et al. 2004) or fully grown, and are, hence, towards the larger end of its size spectrum. However, this is based on the incorrect assumption that the "mature" state and an obliteration of most braincase sutures, noted for the Giganotosaurus holotype (Coria and Currie 2003), are evidence of a senescent growth stage. In fact, a determinate growth model may not even be the universal state for dinosaurs (Myhrvold 2013), rendering comparisons of "fully grown" individuals pointless when it is not a given that both taxa in question actually ever stopped growing. Even more importantly to this specific case, the obliteration of cranial sutures is not a reliable indicator of maturity in archosaurs: Bailleul et al. (2016) demonstrated that in Alligator, the only cranial sutures that are obliterated do so during embryonic development, while the other sutures in fact open up (rather than fuse) as the animal grows. Without detailed knowledge of the ontogenetic development of Giganotosaurus itself, the state of suture fusion on a small part of its skull is effectively useless for ascertaining its relative state of maturity, and it is not at all known if it is more appropriate to compare the available Giganotosaurus specimens to young and actively growing, or old and fully grown adults of T. rex. In the absence of such knowledge, a simultaneous comparison to both (i.e. a pooled sample of all adult T. rex individiduals) is the only option that objectively accounts for all possibilities without preferring one over the other. While it is, of course, true that the absence of information on the precise growth stage and trajectory of Giganotosaurus introduces additional uncertainty, it does not change the basic assessment of which taxon is more likely to be larger.
In conclusion, the available evidence suggests that, as a strong tendency, Giganotosaurus carolinii was probably larger than Tyrannosaurus rex. While this size difference is not statistically significant at p<0.05, it is approximately three times as probable as the reverse. Even though future study of the known material (particularly of the histology and ontogeny, but also osteology of Giganotosaurus) and the recovery of more material (particularly of Giganotosaurus) do hold the potential of changing this verdict, as of now it does meet and exceed criteria commonly applied in vertebrate paleontology in order to establish differences in size or other quantitative parameters. It also supports the statements by Persons et al. (2019) as well as earlier works (e.g. Mazzetta et al. 2004, Calvo and Coria 1998) that pointed out the probable greater size of Giganotosaurus as compared to T. rex (in spite of some methodological shortcomings or vagueness in some of these studies). As of now, it appears that at least if one wants to proclaim a largest known theropod, it should be Giganotosaurus.
–––References:
Bailleul, A.M., Scannella, J.B., Horner, J.R., and Evans, D.C. 2016. Fusion Patterns in the Skulls of Modern Archosaurs Reveal That Sutures Are Ambiguous Maturity Indicators for the Dinosauria. PLOS ONE, 11:e0147687. https://doi.org/10.1371/journal.pone.0147687.
Bates, K.T., Manning, P.L., Hodgetts, D., and Sellers, W.I. 2009. Estimating mass properties of dinosaurs using laser imaging and 3D computer modelling. PloS one, 4:e4532. https://doi.org/10.1371/journal.pone.0004532.
Bruñén, F. 2016a. [online]. Tyrannosaurus rex skeletal diagram (FMNH PR 2081). DeviantArt. Retrieved February 27, 2025, from https://www.deviantart.com/franoys/art/Tyrannosaurus-rex-skeletal-diagram-FMNH-PR-2081-640670460.
Bruñén, F. 2016b. [online]. Giganotosaurus carolinii skeletal diagram. DeviantArt. Retrieved February 27, 2025, from https://www.deviantart.com/franoys/art/Giganotosaurus-carolinii-skeletal-diagram-645266194.
Bruñén, F. 2017. [online]. Tyrannosaurus rex size. by Franoys on DeviantArt. DeviantArt. Retrieved February 28, 2025, from https://www.deviantart.com/franoys/journal/Tyrannosaurus-rex-size-682386614.
Calvo, J.O. and Coria, R. 1998. New specimen of Giganotosaurus carolinii (Coria & Salgado, 1995), supports it as the largest theropod ever found. Gaia, 15:117---122.
Campione, N.E. 2020. MASSTIMATE: body mass estimation equations for vertebrates. R. Retrieved from https://CRAN.R-project.org/package=MASSTIMATE.
Campione, N.E., Evans, D.C., Brown, C.M., and Carrano, M.T. 2014. Body mass estimation in non-avian bipeds using a theoretical conversion to quadruped stylopodial proportions. Methods in Ecology and Evolution, 5:913---923. https://doi.org/10.1111/2041-210X.12226.
Coria, R.A. and Currie, P.J. 2003. The braincase of Giganotosaurus carolinii (Dinosauria: Theropoda) from the upper cretaceous of Argentina. Journal of Vertebrate Paleontology, 22:802---811.
Coria, R.A. and Currie, P.J. 2006. A new carcharodontosaurid (Dinosauria, Theropoda) from the Upper Cretaceous of Argentina. Geodiversitas, 28:71---118.
Coria, R.A. and Salgado, L. 1995. A new giant carnivorous dinosaur from the Cretaceous of Patagonia. Nature, 377:224. DOI: 10.1038/377224a0.
Erickson, G.M., Makovicky, P.J., Currie, P.J., Norell, M.A., Yerby, S.A., and Brochu, C.A. 2004. Gigantism and comparative life-history parameters of tyrannosaurid dinosaurs. Nature, 430:772.
Folkes, D. 2023. [online]. The LARGEST theropod dinosaur known to science…. THECODONTIA. Retrieved February 27, 2025, from https://www.thecodontia.com/blog/the-largest-theropod-dinosaur-known-to-science.
Hartman, S.A. 2013. [online]. Mass estimates: North vs South redux. Dr. Scott Hartman’s Skeletal Drawing.com. Retrieved August 16, 2023, from https://www.skeletaldrawing.com/home/mass-estimates-north-vs-south-redux772013.
Hutchinson, J.R., Bates, K.T., Molnar, J., Allen, V., and Makovicky, P.J. 2011. A computational analysis of limb and body dimensions in Tyrannosaurus rex with implications for locomotion, ontogeny, and growth. PLoS One, 6:e26037. https://doi.org/10.1371/journal.pone.0026037.
Larramendi, A., Paul, G.S., and Hsu, S. 2021. A review and reappraisal of the specific gravities of present and past multicellular organisms, with an emphasis on tetrapods. The Anatomical Record, 304:1833---1888. https://doi.org/10.1002/ar.24574.
Larson, N.L., Larson, P.L., and Carpenter, K. 2008. One hundred years of Tyrannosaurus rex: the skeletons. Tyrannosaurus rex, the tyrant king, 1:56. Indiana University Press, Bloomington, IN.
Larson, P.L. 2008. Variation and sexual dimorphism in Tyrannosaurus rex. Tyrannosaurus rex: The Tyrant King, 103---130.
Mallon, J.C. and Hone, D.W.E. 2024. Estimation of maximum body size in fossil species: A case study using Tyrannosaurus rex. Ecology and Evolution, 14:e11658. https://doi.org/10.1002/ece3.11658.
Mortimer, M. 2025. [online]. Tyrannosauroidea. The Theropod Database. Retrieved February 28, 2025, from https://theropoddatabase.github.io/Tyrannosauroidea.html#Tyrannosaurusrex.
Mazzetta, G.V., Christiansen, P., and Fariña, R.A. 2004. Giants and bizarres: body size of some southern South American Cretaceous dinosaurs. Historical Biology, 16:71---83. https://doi.org/10.1080/08912960410001715132.
Myhrvold, N.P. 2013. Revisiting the estimation of dinosaur growth rates. PloS one, 8:e81917.
Naturalis. 2018. Tyrannosaurus rex Trix in our museum. Sketchfab. Retrieved February 21, 2025, from https://sketchfab.com/models/67e4573e2fe849f4958bb702797b938e/embed?autostart=1.
Paes, H. 2020. [online]. Tyrannosaurus rex skeletal reconstruction. DeviantArt. Retrieved February 28, 2025, from https://www.deviantart.com/randomdinos/art/Tyrannosaurus-rex-skeletal-reconstruction-831025948.
Paul, G.S., Persons, W.S., and Van Raalte, J. 2022. The Tyrant Lizard King, Queen and Emperor: Multiple Lines of Morphological and Stratigraphic Evidence Support Subtle Evolution and Probable Speciation Within the North American Genus Tyrannosaurus. Evolutionary Biology, 49:156---179. DOI: 10.1007/s11692-022-09561-5.
Paul, G.S. 2024. The Princeton Field Guide to Dinosaurs. Princeton University Press.
Persons, W.S., Currie, P.J., and Erickson, G.M. 2020. An older and exceptionally large adult specimen of Tyrannosaurus rex. The Anatomical Record, 303:656---672. https://doi.org/10.1002/ar.24118.
Snively, E., O’Brien, H., Henderson, D.M., Mallison, H., Surring, L.A., Burns, M.E., Jr, T.R.H., Russell, A.P., Witmer, L.M., Currie, P.J., Hartman, S.A., and Cotton, J.R. 2019. Lower rotational inertia and larger leg muscles indicate more rapid turns in tyrannosaurids than in other large theropods. PeerJ, 7:e6432. PeerJ Inc. https://doi.org/10.7717/peerj.6432.
Therrien, F. and Henderson, D.M. 2007. My theropod is bigger than yours… or not: estimating body size from skull length in theropods. Journal of Vertebrate Paleontology, 27:108---115. https://doi.org/10.1671/0272-4634(2007)27[108:MTIBTY]2.0.CO;2.
SpinoInWonderland. 2022. [online]. Volumetric estimate for Giganotosaurus. The Sauropodomorph’s Lair. Retrieved February 27, 2025, from https://thesauropodomorphlair.wordpress.com/2022/01/09/volumetric-estimate-for-giganotosaurus/.
SpinoInWonderland. 2023. [online]. Giganotosaurus carolinii skeletal reconstructions. DeviantArt. Retrieved February 27, 2025, from https://www.deviantart.com/spinoinwonderland/art/Giganotosaurus-carolinii-skeletal-reconstructions-997618549.